Chromo phore |
Exam ple |
Excit ation |
λmax, nm |
ε |
Solvent |
C=C |
Ethene |
π __> π* |
171 |
15,000 |
hexane |
C≡C |
1-Hex yne |
π __> π* |
180 |
10,000 |
hexane |
C=O |
Ethanal |
n __> π*
π __> π* |
290
180 |
15
10,000 |
hexane
hexane |
N=O |
Nitro methane |
n __> π*
π __> π* |
275
200 |
17
5,000 |
ethanol
ethanol |
C-X X=Br
X=I |
Methyl bromide
Methyl Iodide |
n __> σ*
n __> σ* |
205
255 |
200
360 |
hexane
hexane |
|
From the chart above it should be clear that the only molecular moieties
likely to absorb light in the 200 to 800 nm region are pi-electron functions and
hetero atoms having non-bonding valence-shell electron pairs. Such light
absorbing groups are referred to as chromophores. A list of some simple
chromophores and their light absorption characteristics is provided on the left
above. The oxygen non-bonding electrons in alcohols and ethers do not give rise
to absorption above 160 nm. Consequently, pure alcohol and ether solvents may be
used for spectroscopic studies.
The presence of chromophores in a molecule is best documented by UV-Visible
spectroscopy, but the failure of most instruments to provide absorption data for
wavelengths below 200 nm makes the detection of isolated chromophores
problematic. Fortunately, conjugation generally moves the absorption maxima to
longer wavelengths, as in the case of isoprene, so conjugation becomes the major
structural feature identified by this technique.
Molar absorptivities may be very large for strongly absorbing chromophores
(>10,000) and very small if absorption is weak (10 to 100). The magnitude ofε
reflects both the size of the chromophore and the probability that light of a
given wavelength will be absorbed when it strikes the chromophore.
4. The Importance of Conjugation
A comparison of the absorption spectrum of 1-pentene, λmax = 178
nm, with that of isoprene (above) clearly demonstrates the importance of
chromophore conjugation. Further evidence of this effect is shown below. The
spectrum on the left illustrates that conjugation of double and triple bonds
also shifts the absorption maximum to longer wavelengths. From the polyene
spectra displayed in the center diagram, it is clear that each additional double
bond in the conjugated pi-electron system shifts the absorption maximum about 30
nm in the same direction. Also, the molar absorptivity (ε) roughly doubles with
each new conjugated double bond. Spectroscopists use the terms defined in the
table on the right when describing shifts in absorption. Thus, extending
conjugation generally results in bathochromic and hyperchromic shifts in
absorption.
The appearance of several absorption peaks or shoulders for a given chromophore
is common for highly conjugated systems, and is often solvent dependent. This
fine structure reflects not only the different conformations such systems may
assume, but also electronic transitions between the different vibrational energy
levels possible for each electronic state. Vibrational fine structure of this
kind is most pronounced in vapor phase spectra, and is increasingly broadened
and obscured in solution as the solvent is changed from hexane to methanol.
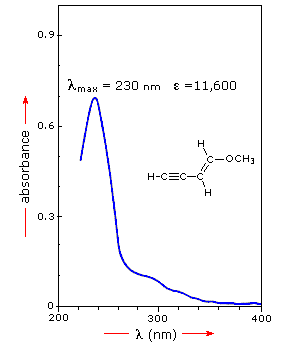
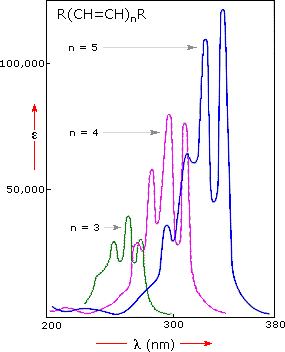
Terminology for Absorption Shifts
Nature of Shift |
Descriptive Term |
To Longer Wavelength |
Bathochromic |
To Shorter Wavelength |
Hypsochromic |
To Greater Absorbance |
Hyperchromic |
To Lower Absorbance |
Hypochromic |
|